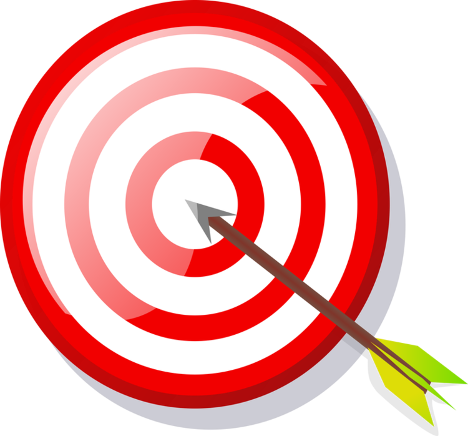
https://www.goodfreephotos.com
Most wineries manage SO2 by measuring free SO2, then determining an addition rate to achieve a specific target. But how should that target be determined? Sulfur dioxide is used in wine as an antioxidant and an antimicrobial agent. Each form of SO2 in solution contributes to the antioxidant function. The molecular form will react with H2O2, quenching a cascade of oxidation set off when phenols are present. The bisulfite form binds with quinones, also quenching this cascade as well as inactivating oxidizing enzymes, the fastest way to browning. The sulfite form reacts with oxygen directly, but slowly (1,2).
The antimicrobial properties, however, are mostly due to the molecular form, which is why this is the focus of most SO2 management decisions. Several studies have been done to determine the rate of SO2 needed to inhibit or kill microbes. Often these rates are reported as ranges because the effective dose is dependent on other factors, such as the alcohol and pH of the wine, or the stage of growth of the microbe. For example, yeast are more susceptible to SO2 in the early stages of population growth than in the later stages (2). Also, care must be taken as some levels are reported to inhibit growth, but those microbes may still be present in the wine in a dormant state that will re-animate when SO2 levels fall.
The bisulfite form of sulfur dioxide cannot enter cell membranes but does make a small contribution to microbial stability in two ways. Lactic acid bacteria have been shown to be inhibited by free as well as bound (bisulfide) forms of sulfur dioxide, with as little as 10 mg/L inhibiting growth and 30 mg/L inhibiting viability. Some lactic acid bacteria such as Leuconostic consume acetaldehyde. If the acetaldehyde is bound by bisulfide, this form is then released as SO2 back into solution, which further inhibits growth (1–3). The bisulfite form of sulfur dioxide also binds to thiamine and destroys it. Thiamine is an essential micronutrient for microbes. Destruction of this one micronutrient may limit population growth in spoilage microbes such as Brettanomyces and Lactobacillus. However, overuse of SO2 at crush can limit thiamine for Saccharomyces, and lead to difficulty in primary fermentation (Bruce Zoecklein, personal communication).
When managing SO2 in wine production, free and total sulfur dioxide are the most common measurements used. Free SO2 refers to any form of SO2 in wine that is not bound to another molecule. As mentioned above, the bisulfite form of SO2 binds readily to many common constituents of wine, which also means it is no longer available for use as an antioxidant. Total sulfur dioxide, then, is the sum of the free sulfur dioxide (which includes all unbound molecular, bisulfite and sulfite forms) and bound sulfur dioxide (mostly bisulfite bound to its many targets) (Figure 2)(1,2,4).
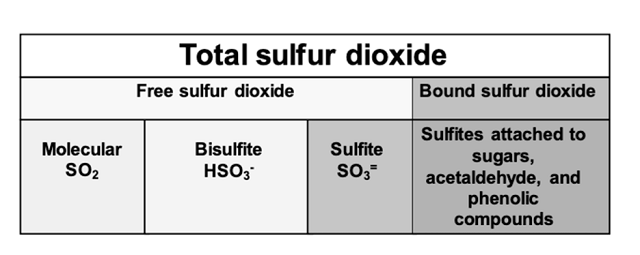
Figure 2: Forms of free and bound sulfur dioxide in wine. From Zoecklein (4)
Since the proportion of SO2 in any one form in wine is pH dependent, the concentration of molecular SO2 can be calculated using the value for free SO2 and the pH of the wine. Many textbooks and online sources provide graphs and tables for this calculation. The Wine Adds website (www.wineadds.com) is a good resource that calculates the molecular sulfur as well as how much SO2 should be added to reach a given target. For example, if a winemaker has a Chardonnay at pH = 3.4 that he/she would like to age at 0.8 ppm SO2, the free SO2 needed to hit this target would be 32 ppm. If that same Chardonnay had a pH of 3.25, only 22 ppm free SO2 would be needed. So, a small acid addition may allow for a much lower SO2 addition. The importance of pH in SO2 management becomes even more pronounced for a Viognier at a pH of 3.6 (50 ppm). To age a Petit Verdot with a pH of 3.8 at a molecular sulfur of only 0.5 ppm, he/she would still need to carry 49 ppm of free SO2!
The most common technique for measuring free sulfur dioxide in the winery laboratory is the aeration oxidation (AO) method. However, there are a few cautions when using this test. In the first step of AO testing, all of the free SO2 is converted to the volatile molecular form by the addition of acid. The volatilized SO2 is then transferred through tubing to a catchment vessel with hydrogen peroxide that converts it into liquid sulfuric acid, which is then titrated with base to determine the total amount of acid. With this method, bisulfite that is loosely bound may be released by the strong acid used in the first step, causing many AO measurements to overestimate the amount of free SO2 in the wine. This is especially true in red wines where bisulfite is bound loosely to anthocyanins, which are released and counted as free SO2 in the analysis but have been shown not to have meaningful activity in the wine (8). If one is using free SO2 measurements by AO to determine molecular SO2 in the wine, the wine is left less protected against microbial spoilage (5).
The attached report gives a summary of target SO2 levels for antimicrobial and antioxidant targets from several sources.
Many guidelines used in winemaking regarding how much SO2 to add to the wine, are based on known quantities needed to inhibit oxidation or microbial growth. Table 1 (insert link) provides a compilation of these quantities from various sources. In many cases, ranges are given rather than firm numbers. Microbes are not a single entity, and different strains will have different tolerance under different conditions. The effectiveness of SO2 on a given population depends on the starting population, so good cellar practices that limit microbes, such as settling after pressing, racking, and filtering, increase overall effectiveness of SO2 additions. Generally, stress is cumulative, so tolerance to SO2 is lower if other stressors such as high ethanol or low pH are also present. SO2 binding also occurs slower in lower pH solutions. However, microbes can acquire tolerance over time. Most targets for antioxidant effects will be met if one is also managing for antimicrobial effects (4).
When determining how much SO2 to add, it is important to keep in mind that some of the added SO2 will be bound up by components of the wine. It is estimated that 1/3 to ½ of the SO2 addition to finished wine will be bound up within the first few days of addition (1,3,6). The proportion is higher in younger wines because more unbound components exist. Botrytis and acetic acid bacteria are both known to produce high levels of compounds that bind SO2, so infected wines will also bind more SO2 (1,7). If the free SO2 is already relatively high, less binding is expected (4). SO2 binding takes time; 3-5 days should be allowed prior to re-testing (4,6). SO2 will be lost faster in barrel storage (up to 5ppm per month) as well as any time the wine is exposed to oxygen (such as during racking, filtration or storage in untopped tanks)(6). When fine tuning SO2 additions for bottling, Zoecklein (3) recommends adding 5-6 ppm to offset the oxygen in the headspace of the bottle while Stamp (6) recommends adding 8-10 ppm to offset all of the processing steps leading up to bottling.
The decision of how much SO2 to add to a wine is a good demonstration of the goldilocks principle: one wants to add just enough but not too little or too much.
Injudicious use can lead to:
- loss of color due to anthocyanin bleaching (8,10)
- reduced rate of tannin polymerization and thus maturation in red wines (9)
- neutralization of other aromas of the wine (1)
- negative odors itself such as a metallic, harsh pungent aroma4, wet wool or burning characteristic (1). Sensory thresholds for these negative aromas depend on the temperature and pH of the wine, with 10ppm in air and 15-40 mg/L in wine common ranges. They are more perceptible in low pH wine at higher temperature (2).
Judicious use can:
- preserve the freshness and fruit character of the wine by protecting it from oxidation (8)
- reverse the nutty, oxidative character of wines caused by acetaldehyde (8)
- increase extraction of color in red wines (9,10)
- prevent browning of white and red wines during aging (2,9,10)
- help prevent microbial spoilage leading to ethyl acetate and acetic acid (8)
- prevent malolactic fermentation in crisp white wines (8)
There is no single right target SO2 for aging wine. Rather, each winemaker must decide the target based on the history, future plans, and winemaking goals for each wine. When deciding on a target free SO2, the best decisions rest on consideration of the disease load of the grapes coming in, the pH and alcohol of the wine, and the amount of time you will be aging the wine before bottling. Other decisions include when to make that first addition, as well as how much to add each time (fewer larger additions or multiple smaller additions).
References
(1)Ribereau-Gayon, P.; Dubourdieu, D.; Doneche, B.; Lonvaud, A. Handbook of Enology Volume 1: The Microbiology of Wine and Vinifications, 2nd ed.; John Wiley & Sons: West Sussex, England, 2006.
(2) Boulton, R.; Singleton, V. L.; Bisson, L. F.; Kunkee, R. E. Principles and Practices in Winemaking; Chapman and Hall, Inc: New York, 1996.
(3) Zoecklein, D. B. Sulfur Dioxide (SO2). Enology Notes Downloads, 16.
(4) Zoecklein, B. W. Sulfur Dioxide: Science behind This Antimicrobial, Antioxidant, Wine Additive. Practical Winery and Vineyard Journal 2009.
(5) Howe, P. A.; Worobo, R.; Sacks, G. L. Conventional Measurements of Sulfur Dioxide (SO2) in Red Wine Overestimate SO2 Antimicrobial Activity. Am J Enol Vitic. 2018, 69 (3), 210–220.
(6) Stamp, C. Methods for Calculating SO2. Wines and Vines 2011.
(7) Margalit, Y. Concepts in Wine Chemistry, 3rd ed.; The Wine Appreciation Guild LTD: San Francisco, California, 2012.
(8) Stamp, C. How Much SO2 to Add and When. Wines and Vines 2011.
(9) Gomez-Plaza, E.; Gil, R.; Lopez-Roca, J.; Adrian, M. Effects of the Time of SO2 Addition on Phenolic Compounds in Wine. Vitis -Geilweilerhof- 2001, 40, 47–48.
(10) Picinelli, A.; Bakker, J.; Bridle, P. V. Model Wine Solutions: Effect of Sulphur Dioxide on Colour and Composition during Ageing; 2015.