Summary
Chardonnay is the most widely planted wine grape variety in Virginia1 and is vinified in many different styles. Two elements that affect Chardonnay style are fermentation yeast and fermentation temperature. In this study, wine made with Ionys WF (Scottlabs) yeast, a yeast strain with properties of acid retention is compared to wine made with CY3079, a classic Chardonnay yeast in broad use. Each yeast strain was tested at cool (15°C) and warm (22°C) ambient temperatures. Ionys WF showed slower fermentation kinetics with greater acid retention. Warm fermentation with Ionys yeast had the lowest total SO2 and lowest volatile acidity at the end of fermentation. In triangle tests comparing CY3079 fermentation at cold and warm temperature, the wines were found to be significantly different. Comparison of wines fermented at warm temperature with different yeasts were also different. Wine fermented with CY3079 at warm temperature received higher scores for body than those fermented cold or with Ionys yeast.
Introduction
Chardonnay is the most planted variety of wine grape in Virginia1 and nearly every winery sells at least one Chardonnay product. Of all the grape varieties, Chardonnay winemaking may be the most varied. There are so many expressions of this grape! Some winemakers choose to treat the juice reductively while others intentionally oxygenate juice. Fermentation vessels include stainless steel, concrete eggs, neutral and new oak barrels. The choice of whether to allow or encourage malolactic fermentation is also different among cellars and among fruit sources. This study explores two other differences in Chardonnay winemaking: fermentation temperature and yeast strain. A primary concern in Chardonnay winemaking in Virginia is the retention of acidity and expression of minerality, so two yeast strains will be tested, one commonly used barrel fermenting yeast and a second that is thought to improve acid retention. This yeast is slow at cool fermentation temperatures, though, so each yeast will be tested at two ambient temperatures, one cooler fermentation in the barrel room (60°F0 and one warmer fermentation in the tank room (72°C). During a panel discussion on Chardonnay winemaking in Virginia at the VWA Technical Meeting in 2017, Jim Law of Linden Vineyards mentioned that he used to ferment his Chardonnay at cool temperatures, but in recent years has moved to a warmer fermentation temperature in this variety. This was further incentive that warm fermentation of Chardonnay should be examined.
Saccharomyces cerevisciae is the primary species of yeast used in commercial wine production. Despite a single domestication event in Mesopotamia around the same time as domestication of wine grapes themselves2, it remains so genetically diverse that no one strain accurately portrays the whole species2. The primary metabolic function of Saccharomyces cerevisciae in wine production is the metabolism of sugar into ethanol with the coupled release of energy. However, complex cellular machinery is also at work to build and maintain cellular components through alternative pathways. It is the work of these pathways that leads to the production of volatile aromas and flavors that gives wine its complexity and diversity3. At any given time, hundreds of substrates are taken up into the yeast cell, transformed by enzymatic pathways, with end products expelled into the environment or used by the yeast cell itself. Though most S. cerevisciae strains share most of these pathways in common, the extent to which any one pathway functions is determined by both genetic differences and environmental influences.3 It is the differences in the functioning and regulation of these enzymes that characterize the myriad of yeast strains that are commercially available for wine production.
Ever since Louis Pasteur first identified S. cerevisciae as the primary fermentative yeast in wine in 1860, many thousands of strains have been isolated, selectively bred and cultured for a wide range of characteristics. Manufacturers of enological products maintain vast collections of yeast strains and produce catalogues complete with descriptions of strain capabilities and tolerances. Regardless of the purpose for which a strain was initially bred, it is wise to heed this advice from Ronald Jackson:
“The main characteristics of most commercial strains are known, but most of their other properties are not, or if known they are buried in research papers not readily accessible to most winemakers. The local conditions often are crucial to feature expressions. It is again up to the winemaker to do their own individual experimentation to determine what best suits their situations and preferences.3”
In this study, two yeast strains will be explored for barrel fermentation of Virginia Chardonnay: CY3079 and Ionys WF.
CY3079 (Scottlabs) is a yeast strain commonly used for barrel fermentation of Chardonnay. Isolated from Burgundy, France, this strain is known for good fermentation kinetics in barrel. It has a temperature tolerance from 59-80°F and is highly recommended for sur lie aging as it autolyzes quickly to release polysaccharides for enhanced mouthfeel in the wine. This yeast enjoys widespread use in Virginia cellars.
According to the manufacturer’s literature, Lallemand Ionys WF yeast (Scottlabs) has been selectively bred to reduce alcohol production and retain acid during red wine fermentations. This yeast strain produces organic acids such as succinate during fermentation and has been shown to increase TA by 0.4 - 1.4 g/L tartaric equivalents. Matthieu Finot has been using this yeast in his barrel fermented Chardonnay for the acid-producing quality. A previous study (2016) did not show large differences in acid chemistry, but did show differences in fermentation kinetics (WF is much slower) as well as sensory differences with a preference for WF fermented wines.
One drawback to using Ionys yeast is that it was bred for fermentation of red wines, which are typically done at warmer temperature. The kinetics for Ionys fermentations at the cooler temperatures commonly used for white wines can be very long (up to four weeks in the previous study). Long fermentation can lead to juice oxidation and loss of reductive volatiles (like thiols) if fermentation takes too long to start and can run the risk of a stuck fermentation as yeast cells languish in a higher alcohol environment3. Also, the growth of epiphytic (non-Saccharomyces) yeast during a slow start may produce spoilage compounds such as acetic acid or ethyl acetate3. One approach to prevent these issues is to allow fermentations to be conducted at warmer temperature. However, fermentation temperature affects many more elements than just rate.
Gene expression in yeast changes in response to ambient temperature during fermentation. Principally, this is thought to allow cell membranes to stay intact in higher temperatures4,5, however these changes also affect enzymatic pathways that produce aroma compounds, both positive and negative3. It is generally thought that fermentation at cooler temperature leads to wines that are fresher and fruitier. This has been confirmed by studies showing higher levels of fruity esters and fatty acid ethyl acetates produced and maintained at cooler temperatures.3 However, the effect of fermentation temperature on the sensory properties of the resulting wine may be a little more complex.
Killian and Ough (1979)6 measured the expression of several esters, compounds that contribute fruity and floral aromas to wine, at fermentation temperatures of 10°C and 15-20°C. They found that some fruity esters (isoamyl acetate, isobutyl acetate, ethyl butyrate, and N-hexyl acetate) were more likely to be expressed at lower temperatures while others (ethyl octanoate, 2-phenethyl acetate) were found in higher concentrations in the warmer fermentation. The esters produced in higher concentration at low temperatures have descriptors such as banana, pear, fruity, current, and apple while those produced more at higher temperatures have descriptors such as rose, honey, fruit, burned and beer. These authors attribute most of the difference in esters in the finished wine to the boiling point of the molecules, indicating loss of volatiles due to evaporation was likely to play a role.
Molina et al (2007)4 measured differences in gene expression during fermentation at 15°C and 28°C and correlated these to differences in concentration of volatiles, showing the differences were due to overall production not just evaporative loss. They used an artificial must containing only glucose, fructose, and amino acids as available substrates and were able to identify 23 volatile fermentation products, indicating yeast transformation of primary metabolites is essential to wine character. They did not find eight other commonly found volatile compounds thought to significantly contribute to wine aroma, indicating grape precursors are also essential4. They also found that ethyl esters were much higher in cooler fermentations (15°C vs. 28°C), but they, too, found positive volatiles that were produced in higher amounts in warmer temperatures. One of these, ethyl-2-methyl butanoate contributes fruity aromas of banana and pineapple, leading to a tropical fruit character to the wine.
Fermentation temperature is also thought to affect the production of thiols. Thiols are sulfur containing compounds7 that can have negative (rotten egg, cooked vegetable) or positive (passionfruit, guava, box tree) aromas. The positive attributes have become principle descriptors for Sauvignon Blanc, but many other varieties have been shown to contain thiols8, including Chardonnay9. At least one study has shown that warmer fermentation temperature produces a higher concentration of thiols10, though these may also be lost to evaporation at a faster rate in warmer fermentations3.
There are other sensory effects of cool fermentations. Often these have higher production of alcohol. Alcohol itself has sensory characteristics (it adds body and can seem sweet in moderate concentrations) and it also affects the perception of other aromatic compounds, making them more volatile11. Slower fermentations have also been shown to have greater complexity due to the activity of non-Saccharomyces yeast, which, in addition to spoilage, can also contribute to highly desirable fruity-floral aromas.
The purpose of this trial is to compare wine chemistry and sensory characteristics of wine fermented with a “standard” yeast (CY3079) and a yeast selected to retain acid in Chardonnay (Ionys WF). Both strains were fermented in duplicate barrels at cool (60 °F ambient) and warm (72 °F ambient) locations.
Methods
With the exception of yeast inoculation and ambient temperature, all winemaking operations were the same among treatments. There were four treatments total:
- CY3079 yeast at “cool” temperature (60°F ambient temp)
- CY3079 yeast at “warm” temperature (72°F ambient temp)
- Ionys WF yeast at “cool” temperature (60°F ambient temp)
- Ionys WF yeast at “warm” temperature (72°F ambient temp)
Chardonnay grapes were harvested on August 29 and chilled overnight, then whole cluster pressed into tank with the addition 20 ppm SO2. After cold settling, on September 1, reverse osmosis was performed on the juice with 13% loss of volume/concentration. Concentrated juice was racked into barrels of comparable age, cooper and dimension (Ana Selection J M 15 or T M 15) in replicate pairs so that there were two barrels per temperature/yeast pair. Juice chemistry was measured before and after RO operation. All barrels received juice from the same tank after reverse osmosis.
Inoculation differences: The initial dose of yeast at inoculation was an additional variable for this trial. Ionys WF is a yeast not usually used in white wines, and based on the experience of the winemaker, this yeast can be slow at lower temperatures. In order to encourage the completion of fermentation, this yeast was inoculated at a rate of 25 g/hL. CY3079 is a strong barrel fermenter that can produce off odors if overly vigorous and, in the experience of the winemaker, produces more pleasant wines when inoculated at 14 g/hL. The decision was made to use different inoculation doses to test the best quality wine produced from each yeast.
Barrels were inoculated with yeast rehydrated in 1.25X Goferm Evolution. For the purpose of the experiment, no acid additions were made. After inoculation, two barrels of each yeast group were placed in the cellar for fermentation (“cool” treatment) while two barrels were placed in the tank room for fermentation (“warm” treatment). It is estimated the cellar was 60°F and the tank room was 72°F during fermentation. Temperature was monitored during fermentation but not otherwise manipulated. Though both yeasts have high nutrient requirements and higher temperatures may increase nutrient utilization, YAN was measured to be 386 ppm, well above that requiring nutrient addition, therefore no nutrient additions were made. Wines were allowed to go through full malolactic fermentation without inoculation prior to the addition of 45 ppm SO2. Wine was aged on lees with battonage once per week beginning when specific gravity dipped below 1 and continuing until sampling for the sensory session.
Sensory analysis was completed by a panel of 29 wine producers. Wines were presented blind in randomly numbered glasses. Tasters were presented with three wines, two of one type and one of another, and asked to identify which wine was different (a triangle test). There were three tasting groups with the unique wine in the triangle test balanced between groups. Tasters were then asked to score each wine on a scale of 0 to 10 for fruit intensity, floral intensity, body and minerality. They were also given open ended questions to describe the wines. Two flights were presented this way, one comparing temperatures for CY3079 barrels and one comparing yeast groups at warm temperature. Results for the triangle test were analyzed using a one-tailed Z test. Descriptive scores were analyzed using repeated measures ANOVA.
A third flight was also presented with all four wines in randomly numbered glasses. The wines were presented in different order for each of three tasting groups. Tasters were asked to sort the wines into two groups of two and give descriptors for why they sorted the way they did. Then tasters were asked to rank the wines in order of preference. Preference rankings were analyzed using a Friedman’s test.
Results
Reverse osmosis treatment increased Brix and TA with little effect on pH (Table 1). The juice used for this trial began with high levels of YAN, indicating any sluggish fermentation was unlikely due to nutrients.
Fermentation kinetics for all barrels are shown in Figure 1. Table 2 summarizes some elements of fermentation kinetics for these wines. Replicate barrels for each treatment showed very similar fermentation kinetics. For each yeast group, fermentation at warm ambient temperature was faster and warmer than that in cool ambient temperature. Ionys fermentation in a warm environment had similar pace of the CY3079 fermentation in the cool environment, but with a warmer maximum temperature (21°C in Ionys warm vs. 17°C in CY cool). For each temperature regime, despite higher inoculation rates, Ionys fermented slower and cooler than CY3079 at the same ambient temperature. Ionys at cool temperature took the longest to complete primary fermentation, finishing near October 3 (a total of four weeks of fermentation). However, the barrels inoculated with CY3079 and fermented warm were the last to finish malolactic fermentation. They were not treated with SO2 until January 29, putting them at risk for oxidation and accumulation of volatile acidity.
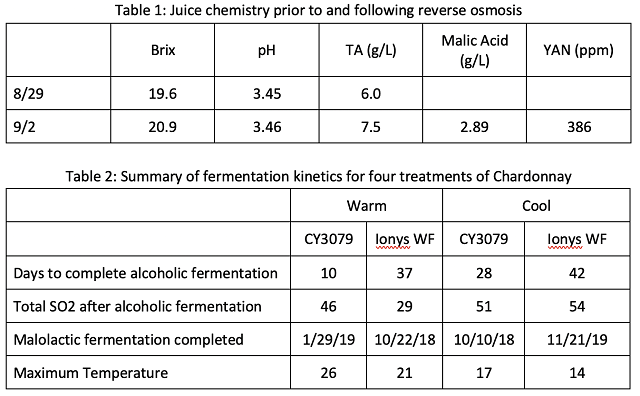
To investigate potential causes of slow malolactic fermentation in the warm CY3079 barrels, free and total SO2 were measured at the end of alcoholic fermentation (Table 3). All total SO2 levels were within a range that is expected to allow malolactic fermentation. Slow malolactic fermentation in these barrels may have been due to high nutrient demand caused by high fermentation temperature leaving little nutrient for malic acid bacteria. The CY3079 barrels fermented at warm temperature also had a higher alcohol than the other barrels (14% compared to 13.4% and below for the other barrels), which may have further inhibited malic acid bacteria. It is unexpected to have a higher level of alcohol in the wine with a warmer fermentation. This may be due to a chaptalization error.
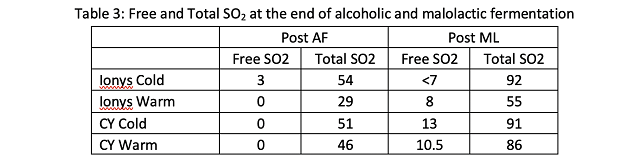
The barrels inoculated with CY3079 and fermented at warm temperature ended malolactic fermentation with notably higher volatile acidity than the other treatments (Table 4), due to the extended period of malolactic fermentation. By contract, the barrels inoculated with Ionys WF and fermented at warm temperature had the lowest volatile acidity at the end of malolactic fermentation. These barrels also finished with the lowest total SO2 and alcohol, and the highest TA. When compared, each Ionys barrel finished with higher acidity than each CY3079 barrel from the same temperature regime, indicating Ionys does help to retain acidity. For all wine chemistry measures, values were very similar in replicate barrels.
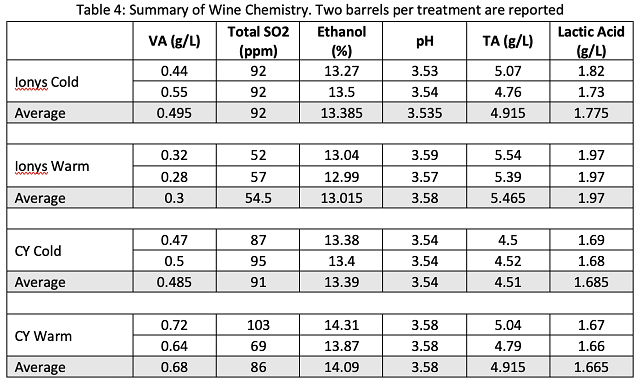
In a triangle test of cool and warm fermented wines inoculated with CY3079, 25 out of 31 respondents were able to distinguish which wine was different, indicating the wines were significantly different (Z=5.84, p< 3x10-5). There were no significant differences in scores for fruit intensity (F=0.91, p=0.34), floral intensity (F=.002, p=0.96), or minerality (F=2.44, p=0.12). Cool fermentations had a small but significantly lower perception of body (F=4.18, p=0.05) with an average of 5.23 (SD=1.42) vs. 5.93 (SD=1.57) for the warmer fermentation. This may be due to the higher alcohol level in the warmer fermentation. When asked what distinguished the wines, several responses included references to “nutty” or oxidized character in the warmer fermentation wine, likely due to its prolonged malolactic fermentation.
In a triangle test of warm fermented wines inoculated with CY3079 vs Ionys WF, 24 out of 31 respondents were able to distinguish which wine was different, indicating the wines were significantly different (Z=5.02, p< 3x10-5). There were no significant differences in scores for fruit intensity (F=0.82, p=0.37), floral intensity (F=.01, p=0.93), or minerality (F=1.66, p=0.2). Fermentation with CY3079 had a small but significantly higher perception of body (F=5.26, p=0.03) with an average of 5.98 (SD=1.23) vs. 5.18 (SD=1.43) for the fermentation with Ionys WF yeast. Once again, this may be due to the higher alcohol level in the warmer fermentation. Several respondents also mentioned a perception of acidity in the Ionys fermentation, which may have diminished the perception of body. Several also mentioned a lactic or oxidized character to the CY3079 fermentation.
When a tasting panel of wine producers was presented with all four wines in randomly numbered glasses and asked to sort them into two groups of two, they were most likely to be sorted by temperature (Figure 2), indicating that temperature was more important than yeast group in sensory characteristics of the wine. However, 9 responders sorted in a way that reflected neither yeast group nor temperature. There was no significant difference in preference among the wines (Q=1.81, p=0.61).
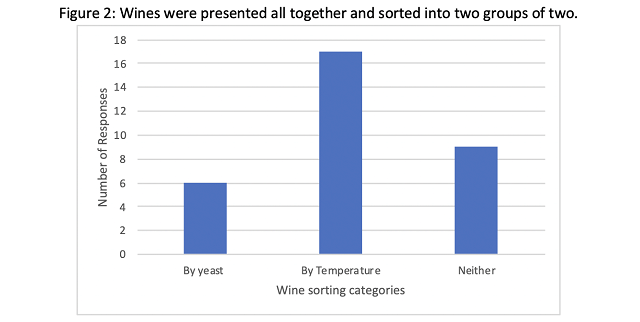
Conclusions
- Increasing the ambient temperature allowed Ionys WF fermentations to proceed more quickly, achieving the same fermentation time as CY3079 fermentations at cooler temperatures.
- Wines produced by Ionys WF yeast had higher total acidity and lactic acid at the end of fermentation than those produced with CY3079 yeast.
- The wine produced with CY3079 yeast at warm temperature had a prolonged malolactic fermentation. It is unclear why this fermentation did not progress well. This wine was perceived as nutty, lactic, and oxidized.
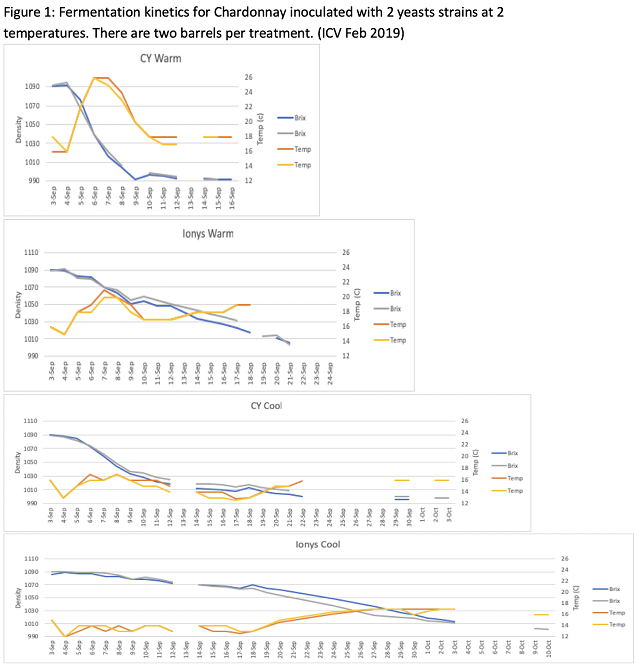
References
(1) Wood, V.; Custer, S.; Watson, K.; Alper, D. Virginia 2018 Commercial Grape Report. 11.
(2) Bisson, L. F. Geographic Origin and Diversity of Wine Strains of Saccharomyces. American Journal of Enology and Viticulture 2012, 63 (2), 165–176. https://doi.org/10.5344/ajev.2012.11083.
(3) Jackson, R. S. Wine Science: Principles and Applications, 4th edition.; Academic Press: Amsterdam, 2014.
(4) Molina, A. M.; Swiegers, J. H.; Varela, C.; Pretorius, I. S.; Agosin, E. Influence of Wine Fermentation Temperature on the Synthesis of Yeast-Derived Volatile Aroma Compounds. Appl Microbiol Biotechnol 2007, 77 (3), 675–687. https://doi.org/10.1007/s00253-007-1194-3.
(5) Torija, M. J.; Beltran, G.; Novo, M.; Poblet, M.; Guillamón, J. M.; Mas, A.; Rozès, N. Effects of Fermentation Temperature and Saccharomyces Species on the Cell Fatty Acid Composition and Presence of Volatile Compounds in Wine. Int. J. Food Microbiol. 2003, 85 (1–2), 127–136. https://doi.org/10.1016/s0168-1605(02)00506-8.
(6) Killian, E.; Ough, C. S. Fermentation Esters — Formation and Retention as Affected by Fermentation Temperature. Am J Enol Vitic. 1979, 30 (4), 301–305.
(7) Waterhouse, A. L. Volatile Thiols. Waterhouse Lab.
(8) Tominaga, T.; Baltenweck-Guyot, R.; Gachons, C. P. D.; Dubourdieu, D. Contribution of Volatile Thiols to the Aromas of White Wines Made From Several Vitis Vinifera Grape Varieties. Am J Enol Vitic. 2000, 51 (2), 178–181.
(9) Capone, D.; Barker, A.; Osidacz Williamson, P.; Francis, I. The Role of Potent Thiols in Chardonnay Wine Aroma: Potent Thiols in Chardonnay Wine. Australian Journal of Grape and Wine Research 2017, 24. https://doi.org/10.1111/ajgw.12294.
(10) Masneuf-Pomarède, I.; Mansour, C.; Murat, M.-L.; Tominaga, T.; Dubourdieu, D. Influence of Fermentation Temperature on Volatile Thiols Concentrations in Sauvignon Blanc Wines. Int. J. Food Microbiol. 2006, 108 (3), 385–390. https://doi.org/10.1016/j.ijfoodmicro.2006.01.001.
(11) Sherman, E.; Greenwood, D. R.; Villas-Boâs, S. G.; Heymann, H.; Harbertson, J. F. Impact of Grape Maturity and Ethanol Concentration on Sensory Properties of Washington State Merlot Wines. Am J Enol Vitic. 2017, 68 (3), 344–356. https://doi.org/10.5344/ajev.2017.16076.